Friday, December 30, 2022
Tuesday, December 27, 2022
Using Nanotechnology to Improve Indoor Air Quality
Emerging research on the use of nanotechnology for increasing indoor air quality (IAQ) has included developments in the removal of indoor air pollutants and volatile organic compounds.
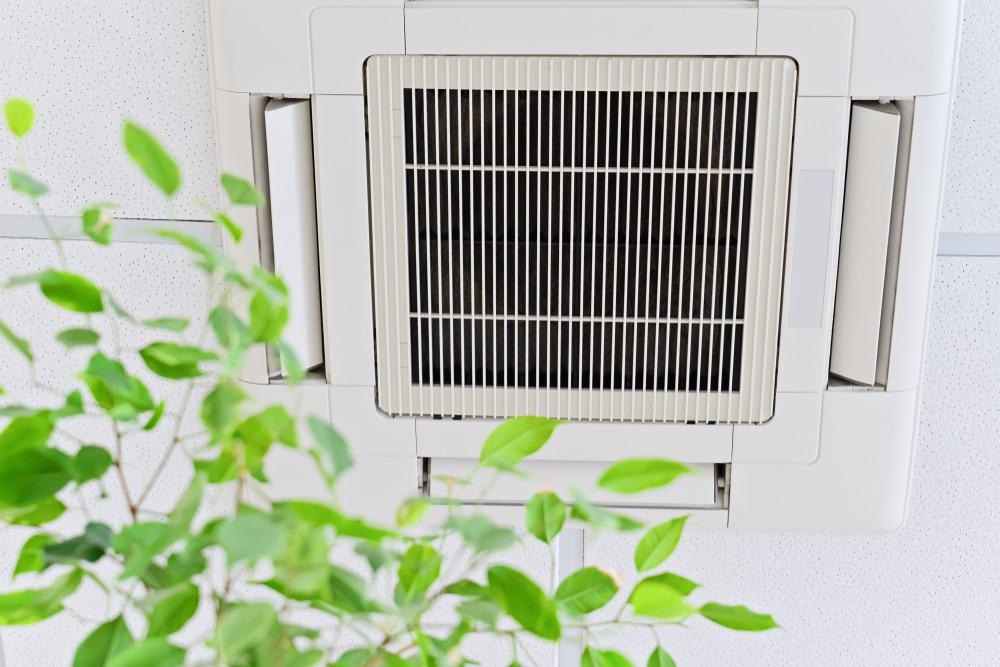
Significance of Indoor Air Quality
Indoor air pollution is one of the top five environmental issues that have been ranked by studies undertaken by the science advisory board within the Environmental Protection Agency (EPA) in the United States.
This type of pollution is a result of burning solid fuel sources, which can consist of firewood, crop waste, or dung for applications such as providing heat and cooking.
The World Health Organization (WHO) has regarded IAQ to be the world’s largest environmental risk as a singular factor, with this type of pollution being responsible for 4.1% of global deaths.
The common pollutants that contribute to IAQ consist of carbon dioxide, nitrogen oxide, volatile organic compounds (VOC), polycyclic aromatic hydrocarbons (PAHs), radon, cigarette smoke, toxic fumes from consumer products and more. These pollutants have been associated with a premature incidence of death as well as resulting in global mortality, especially within low-income countries.
VOCs can be particularly hazardous as these compounds have a boiling point lower than 250 degrees Celsius as well as high vapor pressure, resulting in evaporation at room temperature. Subsequently, when VOCs are present within indoor air, they can cause health issues, from headaches to neck pain and allergic skin reactions.
Additionally, these side effects can be worse when occupants have an existing health condition such as allergies or asthma, which may make these symptoms worse.
Removing VOCs
Removing volatile organic compounds from indoor air has become a critical focal point for researchers due to the high risk these pollutants can have on health.
Technologies like thermal and catalytic oxidation and other processes such as adsorption and filtration have been focused on to aid VOC removal.
Previous research has included the use of photocatalytic oxidation to remove VOCs from indoor air through the use of semiconductor catalysts and ultraviolet light which would aim to convert VOCs from their hazardous state to products such as water and carbon dioxide.
The researchers of this environmentally and health-conscious study have utilized the process of adsorption for the removal of VOCs, with previous studies using adsorption with activated carbon as a common method for addressing these hazardous compounds.
Novel Indoor Air Filtration
The team created multifunctional polyamide-6 (PA-6) electrospun nanofibers for the purpose of filtering indoor air; they utilized nanoclay (NC) as a nanoadsorbent as well as titanium oxide as a nanocatalyst for facilitating the photocatalytic oxidation of toluene.
The use of titanium oxide within nanoparticles has been previously shown to have high photocatalytic activity for toluene as well as being an effective oxidant for VOC decomposition.
Additionally, with electrospun nanofibers gaining popularity in applications such as indoor air filters, their natural inclusion in this research has been apt.
These nanofibers have unique characteristics such as high porosity, as well as having a high surface area to volume ratio, flexibility and modifiable pore size, which have further increased their benefit for use within this study.
The use of polyamide-6 is also quite considered as this polymer has the potential for being an air filter material; its biodegradable nature is also a benefit and with universal use, this research is sustainable in many aspects.
The material also has high mechanical properties such as high tensile strength and chemical stability as well as resistance, which would be helpful when in contact with various pollutants.
The aim of creating these innovative PA-6 electrospun air filters with nanotechnology incorporation would enable the removal of pollutants and increase indoor air quality. Additionally, the PA-6 nanoclay composite electrospun nanofibers are low-cost and allow for long-term use which enables this approach to be more sustainable and advanced when addressing indoor air quality.
Oxidation of toluene with low intensity of UV light can also be enabled, as well as a short reaction time; this would be significant for ensuring air purification is enhanced and stable over a long period of time.
International Conference on Advanced Nanomaterials and Nanotechnology
2nd Edition of NANO | 23-24 Jan 2023 | Delhi, India
Monday, December 26, 2022
NanoComposix Silver Nanopowder Redispersion | Nanotechnology Conferences
International Conference on Advanced Nanomaterials and Nanotechnology
2nd Edition of NANO | 23-24 January 2023| New Delhi, India (Hybrid)
Visit : https://nanotechnology-conferences.sciencefather.com
#Nanocomposites #Nanomagnetism #NanomaterialsforEnergy #Nanobiotechnology #Nanotheranostics #Nanomaterials #Nanotechnology
Thursday, December 22, 2022
Dramatically Improve the Sensitivity of your Lateral Flow Assay with Gol...
International Conference on Advanced Nanomaterials and Nanotechnology
2nd Edition of NANO | 23-24 January 2023| New Delhi, India (Hybrid)
Visit : https://nanotechnology-conferences.sciencefather.com
#Nanocomposites #Nanomagnetism #NanomaterialsforEnergy #Nanobiotechnology #Nanotheranostics #Nanomaterials #Nanotechnology
Monday, December 19, 2022
Laser Ablation and Nanoparticle Generation in Liquid Flow
International Conference on Advanced Nanomaterials and Nanotechnology
2nd Edition of NANO | 23-24 January 2023| New Delhi, India (Hybrid)
Visit : https://nanotechnology-conferences.sciencefather.com
#Nanocomposites #Nanomagnetism #NanomaterialsforEnergy #Nanobiotechnology #Nanotheranostics #Nanomaterials #Nanotechnology
Thursday, December 15, 2022
Nanotechnology Utilized in New Enhanced Oil Recovery Method
A novel enhanced heavy oil recovery approach was presented by integrating a conventional weak alkaline (sodium carbonate) alkaline–surfactant–polymer (ASP) flooding technique with silica nanoparticles.
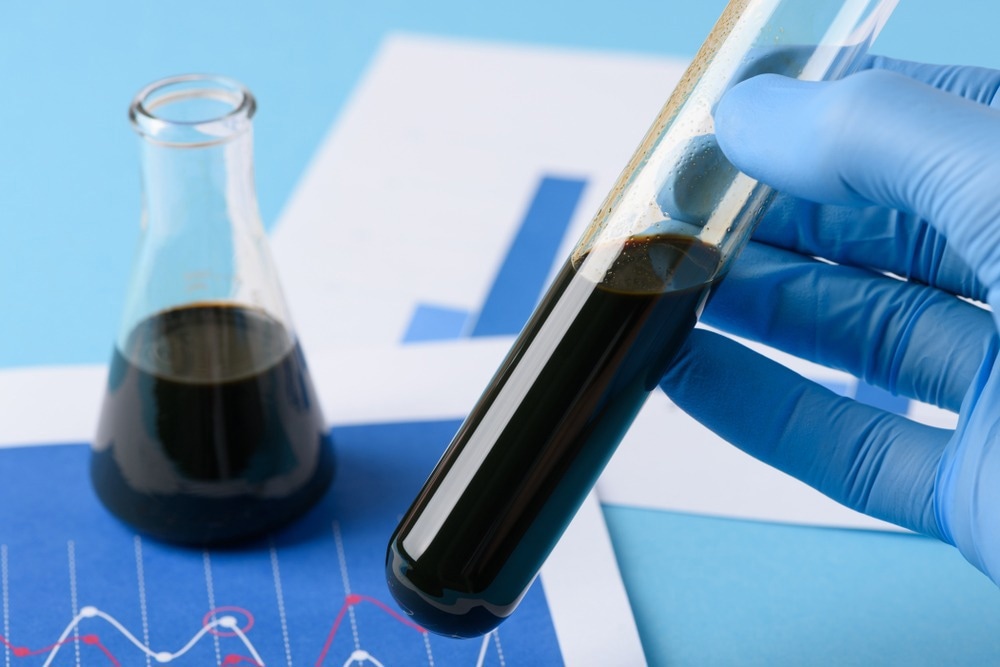
This innovative approach has the potential to improve oil recovery, minimize costs of chemical agents, and eliminate alkali deposits and formation erosion.
The Need for Enhanced Heavy Oil Recovery
With the development of traditional reservoirs in their latter stages, the water content in generated fluids stays large, culminating in a fast drop in reservoir output. After secondary production, fewer than 15% of the natural reserves were retrieved because the undesirable oil-water movement ratio during injection, wettability of reservoirs, and heterogeneous nature.
As a result, it is critical to apply modern technologies to the currently exhausted reservoirs. The use of chemical reagents in this field is thought to be the most effective tertiary method for enhanced heavy oil recovery.
Alkaline-Surfactant-Polymer Methods
Tertiary recovery methods, notably ASP procedures, have proven to be successful chemically enhanced heavy oil recovery processes in certain reservoirs. An ASP technique integrates higher macro-scale swept polymer efficiency as a result of decreased injected water movement with improved micro-scale displacement effectiveness of surfactants (in situ as well as additive saponification).
In contrast with the water injection technique, enhanced heavy oil recovery offered by ASP solution injections technique may be as much as 20% higher.
Nanoparticles in Heavy Oil Recovery Techniques
Combining classical chemical reagents (polymer/surfactant flooding) with nanoparticles has been suggested as an enhanced heavy oil recovery technique.
A polymeric nanofluid is composed of NPs and a polymeric solution. Owing to the lower water-oil movement ratio and the higher displacement oil efficacy, polymeric nanofluids are gaining popularity.
The adsorption of hydrogen bonds and charges among NPs and polymeric molecules boosts the rheological characteristics of the polymeric nanofluid, making it much more resistant to salt and heat.
Research suggests that electrostatic repulsive forces may limit the binding of polymer-grafted hydrophilic NPs on the hydrophobic rock surface. NPs have been shown to boost oil production by modifying the wettability of rock surfaces and lowering interfacial tension.
Limitations of ASP Methods
While ASP processes provide numerous benefits with respect to enhanced heavy oil recovery, higher alkali levels may also produce issues, like alkali scale deterioration, higher expenses, and a significant reduction of the pump-checking cycle.
Addressing these obstacles is crucial for lowering operating costs and enhancing oil recovery effectiveness. Keeping this in view, the team developed a multi-composite flooding approach that combines ASP with silica NPs.
Findings of the Study
The synergistic effects of ASP with silica NPs were carefully investigated using static testing, core displacement testing, and microfluid experiments.
The ASP and ASP/silica solution's interfacial tension values remained low. A mildly alkaline ASP changed the wettability of the rock surface from lipophilicity to weak hydrophilicity. In comparison, the mildly alkaline ASP/silica mixture could quickly convert the neutral wettability of a rock surface to high hydrophilicity.
The recovery of oil improved by 6.67 percent when ASP/silica nanoparticle mixtures were used following water flooding, in comparison with ASP-only solutions. The ASP/silica mixture outperformed the ASP mixture in terms of swept volume and displacement efficiency.
The ASP/silica mixture performed better in emulsification and snap-off compared to a hydrolyzed polyacrylamide/ASP mixture. Droplets of ASP emulsion were more likely to agglomerate, suggesting low thermodynamic stability.
Despite the fact that the ASP/silica droplets collided and distorted extensively, no agglomeration was observed, indicating high thermodynamic, viscoelastic, and kinetic stabilities.
As the silica NPs bound on the emulsion surfaces may act as a deterrent to agglomeration, the emulsion's stability was improved. Greater stability translates to higher microforce acting on a droplet of oil.
Oil droplets were, therefore, more readily pushed and drawn out by emulsion droplets. It showed that the ASP/silica emulsion extracts more oil.
International Conference on Advanced Nanomaterials and Nanotechnology
1st Edition of NANO | 15-16 December 2022 | Dubai, United Arab Emirates (Hybrid)
Cell Sorting by FACS for Sperm | Nanoparticle Bioconjugate Light Scatter...
International Conference on Advanced Nanomaterials and Nanotechnology
2nd Edition of NANO | 23-24 January 2023| New Delhi, India (Hybrid)
Visit : https://nanotechnology-conferences.sciencefather.com
#Nanocomposites #Nanomagnetism #NanomaterialsforEnergy #Nanobiotechnology #Nanotheranostics #Nanomaterials #Nanotechnology
Wednesday, December 14, 2022
What is Ferroelectricity and How Does it Apply Nanotechnology?
The growing attempt to incorporate nanotechnology with electronics has resulted in the realization of emerging conceptual components and devices based on ferroelectric materials, such as transistors, memristors, capacitors, and many others.
What is Ferroelectricity?
Opposite charges are attracted to one another and desire to combine in most materials, including metals. Their only movement is caused by the application of an electric field, which causes them to move to the opposite sides.
However, in ferroelectric materials, an external electric field is not required to maintain the separation of charges, resulting in spontaneous polarization.
The application of an external electric field, on the other hand, has an effect: an electric field in the opposite direction causes the charges to switch sides and reverse the polarization; an electric field in the same direction causes the charges to switch sides and remain polarized. This is why ferroelectric materials are used in a variety of electronic systems, such as those used in medical ultrasounds and radio frequency identification (RFID) cards, among other applications.
There are two variables that represent the fundamental aspect of any ferroelectric: the coercive electric field in correspondence to which the polarization vanishes and the remanent polarization, which represents the ferroelectric's intrinsic polarization when no electric field is applied.
Even when the applied electric field is zero, ferroelectric materials exhibit spontaneous nonzero polarization. The nonlinear nature of ferroelectric materials can be used to create ferroelectric capacitors, which are used in ultrasound machines, infrared cameras, and other applications.
Ferroelectrics are distinguished by the fact that their spontaneous polarization can be reversed by applying a significantly strong electric field in the opposite direction, resulting in a hysteresis loop. This effect can be used as a ferroelectric RAM, computer memory function.
Ferroelectrics are all pyroelectric and piezoelectric, making ferroelectric capacitors extremely useful in sensor applications such as sonar, fire sensors, and vibration sensors.
Materials typically exhibit ferroelectricity only below a certain phase transition temperature, known as the Curie temperature (TC), and are paraelectric above this temperature.
Conventional Ferroelectric Materials
Ferroelectric materials can be structurally classified into three groups. The first is a compound containing hydrogen bonded radicals, in which proton motion is specifically associated with ferroelectric properties. Rochelle Salt and potassium dihydrogen phosphate are two examples (KH2PO4).
The second class of ferroelectric materials consists of ionic crystals with crystal structures similar to perovskite. BaTiO3, KTaO3, NaTaO3, KNbO3, PbTiO3(PZT), lead magnesium niobate (PMN), lead zirconate titanate (PZT), and others are examples.
The third category includes polymers and polymer composites such as PVDF.
Ferroelectricity and its Application in Nanoelectronics
Many applications in nanoelectronics are being driven by the recently discovered two main categories of ferroelectrics. The first is hafnium oxide (HfO2)-based ferroelectrics, which have been extensively researched as a gate dielectric in recent years due to their CMOS compatibility.
The traditional ferroelectrics (such as perovskites and PZT) are not CMOS compatible, and their ferroelectricity tends to disappear when they are thinned to the nanometer scale. HfO2-based ferroelectrics, on the other hand, are ferroelectric to a thickness of 1 nm.
2D ferroelectrics represent the second category. In this regard, graphene (a semimetal) and molybdenum disulfide (MoS2), a semiconductor, are two well-known examples.
HfO2-Based Ferroelectrics: Nanodevices Fabrication and Applications
To reduce the leakage current of billions of transistors integrated in a single chip, the relative dielectric constant must be increased (or relative permittivity). Doping HfO2 with elements such as yttrium and silicon promotes phase transition and the corresponding change in relative permittivity. For example, by doping HfO2 with zirconium oxide during atomic layer deposition, the tetragonal phase with the highest relative permittivity (app. 70) was obtained almost at room temperature.
Tunability of high-frequency components is critical for the advancement of future wireless communications, the Internet of Things and radar technologies. The high coercive fields of HfO2-based ferroelectrics enable massive tunability of microwave devices at only 1-3 V provided by a common battery.
For example, researchers from the National Institute for Research and Development in Microtechnologies Romania, created a microwave filter by growing a 6-nm-thick hafnium oxide doped with zirconium (HfZrO) layer on a 525-nm-thick Si substrate using atomic layer deposition (ALD) techniques. When the applied direct current (DC) voltage is swept between 0 V and 4 V, the filters are continuously tunable within the frequency range 0.1–16 GHz.
Another recently popular concept is the ferroelectric tunnel junction (FTJ), which consists of a nanometer-thick ferroelectric film sandwiched between metal electrodes. The thickness of the ferroelectric layer is thin enough to allow electron tunneling. Researchers designed and built a HfZrO-based tunneling diode with a top electrode made of 5 nm thick Chromium and 100 nm thick gold deposited on a 6-nm thick HfZrO thin film grown directly on doped silicon.
2D Ferroelectric Nanomaterials
Research into 2D ferroelectrics is on the rise thanks to the rapid development of nanotechnology. They could be used to make micro and nano-intelligent parts that have high performance and low energy consumption in the future.
Various 2D vdW materials, such as transition metal dichalcogenides (TMDs), MX2 (M = Mo W, X = Te, Se S), layer perovskites, metal triphosphates, indium selenide (In2Se3), and others, have been reported to be intrinsically ferroelectric; however, only a handful of 2D ferroelectric materials have been confirmed experimentally.
In 2018, researchers from King Abdullah University of Science and Technology reported that layered In2Se3 nanoflakes exhibit low-temperature ferroelectricity. Following that, a graphene/In2Se3 heterojunction was successfully used as a room-temperature ferroelectric diode, and in 2019, a MoTe2 2D nanostructure with ferroelectric properties was discovered at room temperature.
MIT researchers and colleagues recently discovered that the moiré superlattice created by sandwiching two graphene bilayers between atomically thin layers of boron nitride can be ferroelectric as well. One possible application is neuromorphic computation, which is based on circuits containing artificial neurons and synapses that mimic various brain functions like vision and learning.
Future Outlook on Ferroelectric Nanodevices
Ferroelectrics are finding increasing use in low-power nanoelectronics devices such as switches, negative capacitance transistors, and memristors. Although research in this area has just begun, started, real-world applications such as neuromorphic computation, fast processors, and reconfigurable systems for 5G and 6G communications are already significant.
International Conference on Advanced Nanomaterials and Nanotechnology
1st Edition of NANO | 15-16 December 2022 | Dubai, United Arab Emirates (Hybrid)
Nanopesticides: Revolutionizing Sustainable Agriculture
Nanopesticides: Revolutionizing Sustainable Agriculture In the face of growing global challenges such as food security, climate change, and ...

-
Prof. Emad Aly graduated with first honors and excellent degrees from the Department of Mathematics, Ain Shams University . in Egypt. Congra...
-
Using Nanotechnology to Improve Indoor Air Quality Emerging research on the use of nanotechnology for increasing indoor air quality (IAQ) ha...
-
(Nanowerk News) ETH researchers have managed to take three-dimensional pictures of single nanoparticles using extremely short and strong X-r...